Batteries have become ubiquitous in our everyday life, from the vehicles we drive to the personal devices we carry everywhere.
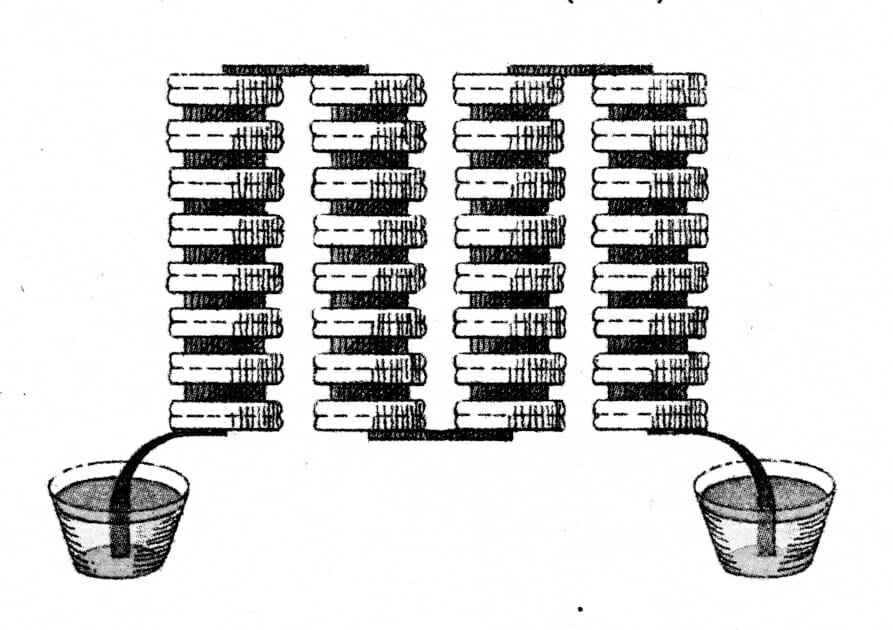
They come in many forms and are almost unrecognisable from their origins in the 1800s.Alessandro Volta built the first battery, which he termed an “electric pile”, using alternating discs of zinc and copper connected by leather or cardboard soaked in salt water. Benjamin Franklin first used the name ‘battery’, which he drew from its military usage, to describe multiple cells connected together and used simultaneously.
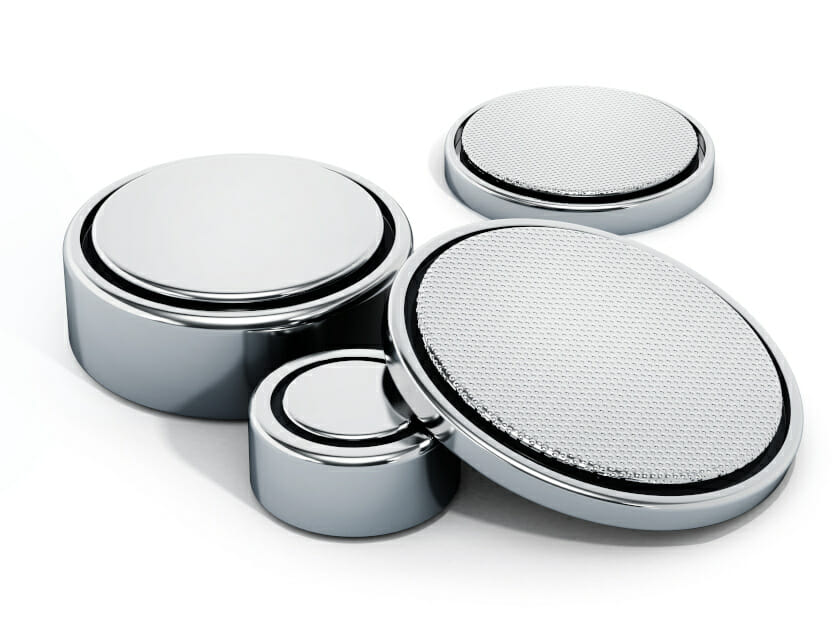
Modern batteries vary enormously, both in their size and in the chemistry that powers them — from vanadium flow batteries, used in micro power generation, to the silver oxide batteries used in watches.
All batteries comprise one or more ‘cells’, an individual unit that generates electricity from the energy produced by chemical reactions. The battery’s application, for example the current and voltage demanded from it, and the required duration of its service will determine the number of cells required and the cell chemistry employed. Historically, single-use (or ‘primary’) batteries were prevalent, with rechargeable (or ‘secondary’) batteries used in certain applications. These days, the huge growth in mobile electronics and increasing awareness of the impact of electronic waste has driven a significant growth in the use of rechargeable batteries.
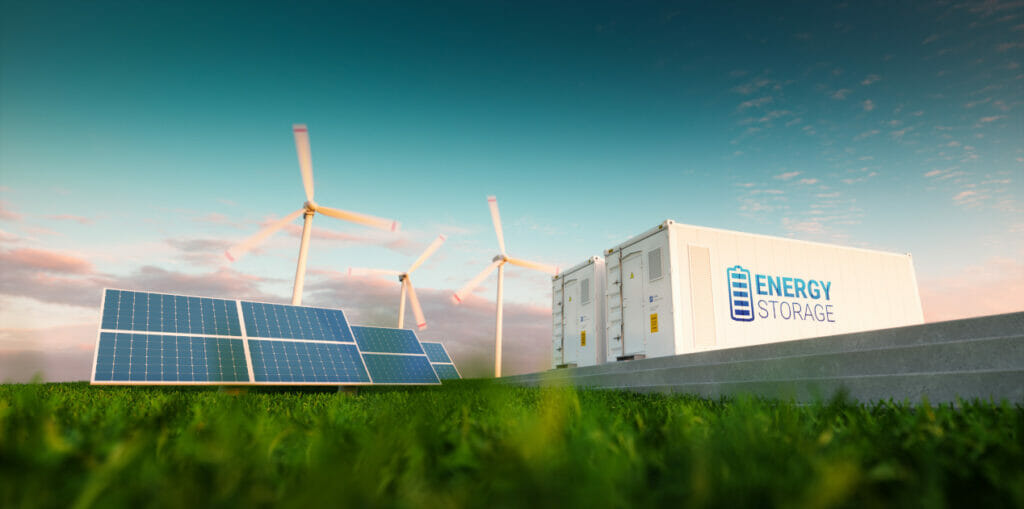
This Insight article will focus on rechargeable ‘lithium ion’ batteries, not to be confused with non-rechargeable (‘primary’) lithium metal batteries. Lithium ion batteries have become one of the most commonly used rechargeable battery technologies. The use of lithium (the lightest metallic element) in batteries is due to its unique chemical and electrochemical properties, which allows the batteries to provide a high energy density for their weight, as well as provide a stable current output across a wide range of charge levels.
CELL CONSTRUCTION
Lithium-ion battery cells are made by bringing together four components:
- A positive electrode (cathode)
- A negative electrode (anode),
- An electrolyte
- A separator
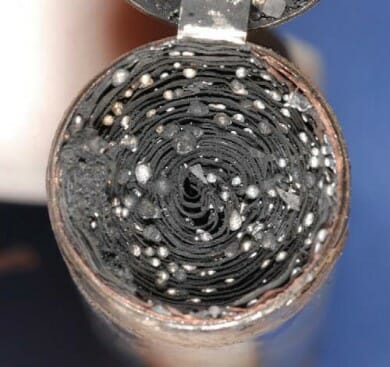
To improve cell performance, the cathode and anode are made with as greater surface area as possible, given the intended size of the final battery assembly. The cathode and the anode are coated in the required surface treatments. Next, the electrolyte and separator are applied between the cathode and anode, and the assembly is then rolled into the relevant shaped package (i.e. a cylinder or a more rectangular ‘prism’ shape).In lithium ion batteries the anodes are generally made from graphite layered on a copper foil collector, with the cathode composed of lithium metal oxide salts (e.g. lithium cobalt oxide – LiCoO2) coated onto an aluminium foil collector. The electrolyte and separator allow the movement of lithium ions between the cathode and anode, to counter the effects of electrons flowing in from or out to the external circuit. Other materials used to coat the cathode and anode can modify the stability and performance of the cell. The result of well-matched cell chemistry is that lithium ion cells normally operate safely in the voltage range of 3.0 to 4.2 V.
The physical forms of the cells are many and varied, and will often depend on the end application in which they will be used. Most lithium ion cells are formed by rolling the layers together, in a similar manner to a paper roll, which allows a more efficient use of available space, and provides mechanical robustness. The most common size of cylindrical design has dimensions of approximately 18 mm diameter and 65 mm length; so called ‘18650’ cells. These battery cells are used in many applications such as laptop computers and electric cars. For example, Tesla have employed thousands of 18650 cells in their car batteries. Other cell shapes include ‘soft’ oblong pouches or ‘hard’ prismatic shapes, and these are used in more niche applications, e.g. mobile phones and cameras.
Whichever form of construction is used, there are numerous reports on the failures of lithium ion batteries. Some of the failures are due to clear misuse (e.g. from physical impact), but others have arisen from problems with poor manufacture, or incorrect storage.
FAILURE MECHANISMS
Lithium ion batteries are exceptionally useful due to their high energy capacity in a lightweight/compact volume that can provide an almost constant output voltage regardless of the power required in the end application. However, this comes at the cost of their robustness in certain circumstances. Batteries in general can suffer failures either catastrophically, or otherwise. The high energy density of lithium-ion cells, combined with the nature of their constituent materials, can often result in catastrophic failures and these incidents are of particular interest to forensic investigators because of the consequential fires or personal injuries.
Catastrophic failure of cells can be initiated in a number of different ways such as thermal runaway or mechanical faults. Thermal runaway occurs when the heat generated by an operating cell cannot be dissipated into the environment, increasing its temperature and affecting internal reactions. This leads to further heating of the cell and further rapid reactions until the cell fails, often with an uncontrolled release of the vaporised electrolyte, which is normally a flammable, organic liquid. The electrolyte in its gaseous form can then be ignited by the high temperature of the surrounding cell, and cause the consequential ignition of any nearby combustible materials.[i]
Thermal runaway can also be initiated when a cell is exposed to a high ambient temperature, for example, within a burning room. Thermal runaway can also result from changes in the cell structure and chemistry that leads to decomposition reactions brought about during charging or discharging, and internal short circuits.
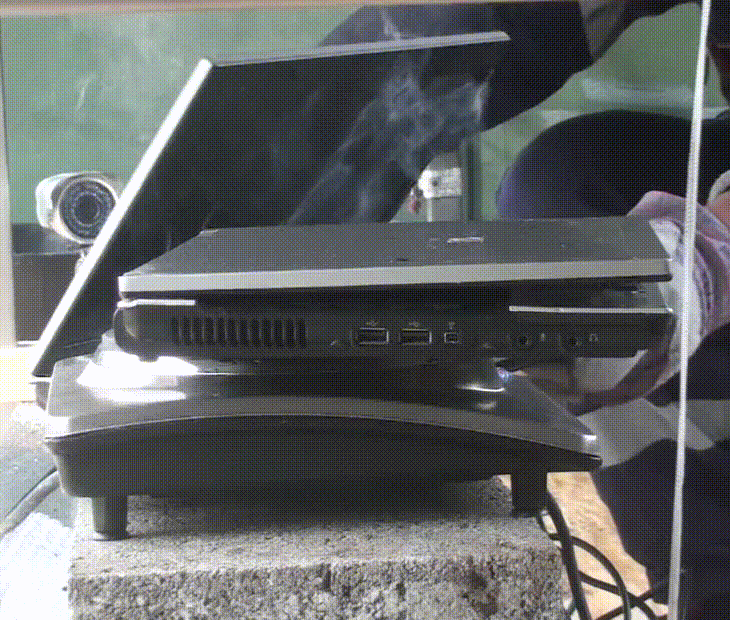
Other causes of catastrophic cell failure can result from mechanical faults in the cell leading to short-circuits that cause a rapid, localised build-up of heat. For example, the 2016 recall of some 2.5 million Samsung smartphone devices was issued because of two principal mechanical faults in the cell construction.[ii]
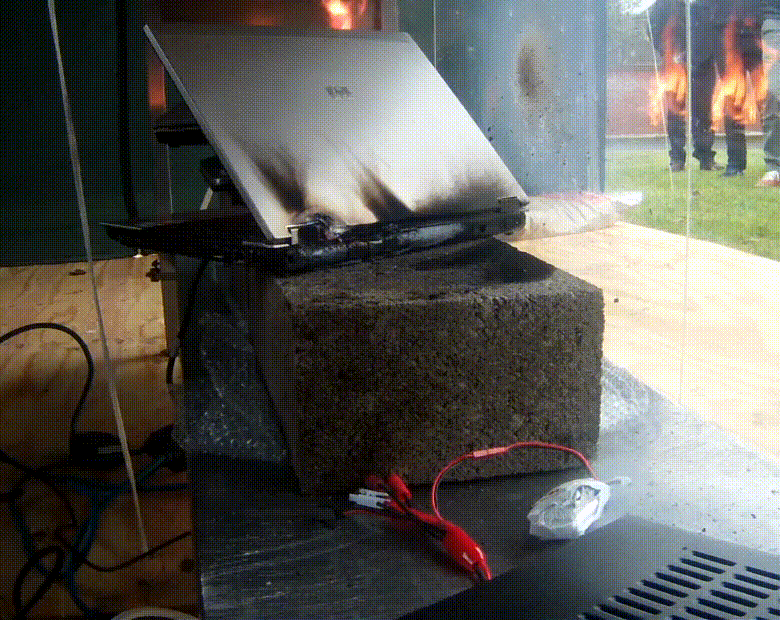
The first led to contact (i.e. a short circuit) between cell electrodes because of the pressure on the pouch cell as it underwent slight changes in volume during charge and discharge. The second fault occurred where the cell’s electrical connection tabs were welded to the positive electrode. The weld formed a high burr (a ‘sharp’ formation) that could eventually cut through the layers in the cell, forming a short circuit between the electrodes.
Mechanical failures also occur from gross damage to cells, for example from puncturing a battery with a sharp object. Hawkins has investigated fires where animals have bitten cells, which likely resulted in release of the electrolyte and potentially a short circuit. Other sharp objects that can cause similar damage to cells include screws, nails, and heavy blunt objects. Such damage does not lead to immediate failure; there can be a short delay, or failure might not occur until the damaged cell is subsequently charged.
In batteries where there are multiple cells, the catastrophic failure of one cell can lead to a more energetic failure of the neighbouring cells, to the point where the whole battery can explode.
Lithium ion batteries have a safe operating charge state between about 20% and 100% of their designed full charge. If the cells are charged to above 100%, a breakdown at both the anode and the cathode can occur. At the anode (negative electrode) over-charging can result in dendritic structures of metallic conductive lithium, which leads to short circuits. At the cathode, other chemical reactions can occur, which cause exothermic failure of its crystalline structure. Lithium metal can also react directly with the electrolyte to generate heat and flammable gases. It is not uncommon for over-charging to cause both thermal runaway and catastrophic failure of the cell (or cells).
Conversely, over-discharge occurs when the cell voltage falls below a lower threshold (i.e. 3.0 V) and moves toward 0 V. If a lithium ion cell is discharged below the lower threshold then damage to electrodes can result from the formation of metallic lithium. Well designed and built lithium ion batteries incorporate electrical circuits designed to limit the potential for either over-charge or over-discharge. However, such circuit protections do not always prevent over-discharge. For example, the protection circuit might limit discharge to the lower threshold voltage, but then on long-term storage the cell can self-discharge below that threshold. The protection circuits generally do not prevent charging once the cell voltage has reduced below a safe operating level. Subsequent charging of an over-discharged cell can then lead to catastrophic failure of the cell.
As lithium ion batteries have become more common, and the demand for production has increased, there are an increasing number of cells being manufactured that use lower quality materials and production practices, to provide a lower cost product. Poorer quality cells are more prone to failures, for example from the entrapment of foreign objects in the cell during manufacturing, which can lead to catastrophic failures during use. The introduction of ‘wrinkles’ as the cell is wrapped can also lead to faults, such as those experienced in the large lithium ion battery systems of the Boeing 787 Dreamliner, as reported by the United States National Transportation Safety Board (NTSB) in 2013.[iii] During the initial few charges of a new cell, the cell dimensions can change as the cell is ‘formed’. Any foreign objects or cell defects can cause catastrophic cell failure in the first few charge cycles. Controlled cell formation is usually part of the manufacturing process, and is intended to capture faulty cells before they leave the factory. Quality control checks on batteries can also comprise several charge/discharge cycles to reduce the likelihood of consumers receiving defective products.
A recent illustration of problems with over-charging and over-discharging are the issues around electronic cigarettes. In these devices, the charge protection circuits can be absent from the cigarette component and, if a generic charger connection is used (i.e. one not provided by the manufacturer of the cigarette) can also allow the device to be charged in an unregulated fashion, using an incompatible charger which uses incorrect charging voltages and current. This results in over-charge of the device and catastrophic failure of its cells.
FORENSIC ANALYSIS
From a fire investigator’s perspective, fires resulting from lithium batteries can be challenging to investigate due to the fragile condition of batteries and their cells after an incident. Rapid attendance of a fire investigator increases the prospect of retaining forensic evidence that might permit the causative fault, or origin of the fire, to be identified. In Hawkins’ experience, the most common remains of batteries following a fire will be the copper foil electrodes, some other minor metallic components, and small circuit boards – all of which are easily lost or damaged upon disturbance of the fire scene. If these can be recovered then, in some cases, there can be sufficient evidence to provide witness to thermal events that occurred before the fire. In other cases, CCTV can provide clearer evidence as to the origin of the fire. Vigilance is needed to safeguard the delicate nature of the evidence when lithium ion batteries are a potential cause of fires so as to retain the best chances of a successful forensic investigation. A prompt forensic examination of a fire scene ensures the best chance of determining the cause of a fire involving lithium ion batteries, or a near miss.
Non-destructive testing, such as x-ray analysis, of a battery provides useful information about the cell structure, damage within the core of the cell, and the presence of any protective devices and their operation, without the need for a potentially destructive physical examination. Given how severely damaged cells or batteries can be after a fire (whether or not they were the cause) the retention of exemplar batteries and cells is invaluable in determining the design, construction and quality of the batteries involved.
This article has focussed on the many means by which lithium ion rechargeable batteries can fail. However, it is worth noting that such failures should be put in the context that for the large number of applications, and very large number of physical cells currently in use, the failure rates (particularly incendive failures) are very low.
Lithium-ion battery design is a rapidly evolving field, with alternative cell chemistries and materials being researched to provide enhanced capacity, faster charging or discharging, or greater safety during use. Significant investment in research has progressed, and continues to progress the knowledge about lithium ion batteries, along with the improvements in their chemistries that will provide safer and more stable performance in the future.
ABOUT THE AUTHORS
Jake Irwin is a Chartered Chemist (CChem) and Chartered Scientist (CSci) with a background in analytical and organic chemistry. Joining Hawkins in 2012, Jake brought his prior experience in criminal forensics into the investigation of fires, explosions, and chemical incidents. This work has involved a number of investigations relating to fires that have resulted from lithium ion battery failures.
Richard Heath is a Chartered Engineer (CEng) with a background in Electronic and Electrical Engineering. He has worked for Hawkins since 2004, and has investigated a diverse range of cases, involving product failures, personal injuries and fires, providing advice to insurers, solicitors, and private companies in relation both civil and criminal incidents.